What is a Pulsar?
Today, we are going to pick up a topic that we briefly touched on in one of the previous Science Capsules: the Neutron Star. However, we are not going to talk about just any Neutron Star. No, instead, we will focus on one particularly interesting case, the Pulsar. But what exactly is a Pulsar?
As I alluded to before, the Pulsar is a special type of Neutron Star. Much like its other counterparts, this celestial body is much smaller (about 20 km) than a regular star. Even so, it actually contains even more mass than our own Sun. This leads to a density that is much, much greater than that of basically any other celestial body, excluding Black Holes, of course. Another quintessential characteristic is that a Pulsar emanates an incredibly strong magnetic field. In fact, the strength of a Pulsar’s magnetic field can range anywhere from 100 million times to 1 quadrillion times (!) that of Earth. The final trait, and this is what really sets it apart from other Neutron Stars, is the way it appears to be releasing light at fixed intervals, kind of like a pulse; hence the name, Pulsar.
How Does a Pulsar Star Form?
To fully understand why a Pulsar has these incredible characteristics, it is important to look at how it is formed. This ties back to the topic from the aforementioned Science Capsule, “The End States of Stars”. There, we discussed how different stars “die”. In the case of stars that are between 1.5 and 3 times the mass of the Sun, a supernova will occur after the star runs out of elements to fuse, much like it would in stars larger than 3 times the mass of the Sun. However, while what is left behind by those even more massive stars is a Black Hole, stars in the range of 1.5-3 Solar Masses will, instead, leave a Neutron Star.
Just as is the case with a Black Hole, these Neutron Stars are very dense, due to the collapse of the star’s mass under the pressure of its own gravity. In the case of Pulsars, they also will be producing the massive magnetic fields mentioned before. This will then lead to the defining trait we discussed above, the emission of light in specific time intervals.
And speaking of, now is probably a good time to talk about how exactly these “pulses” happen.
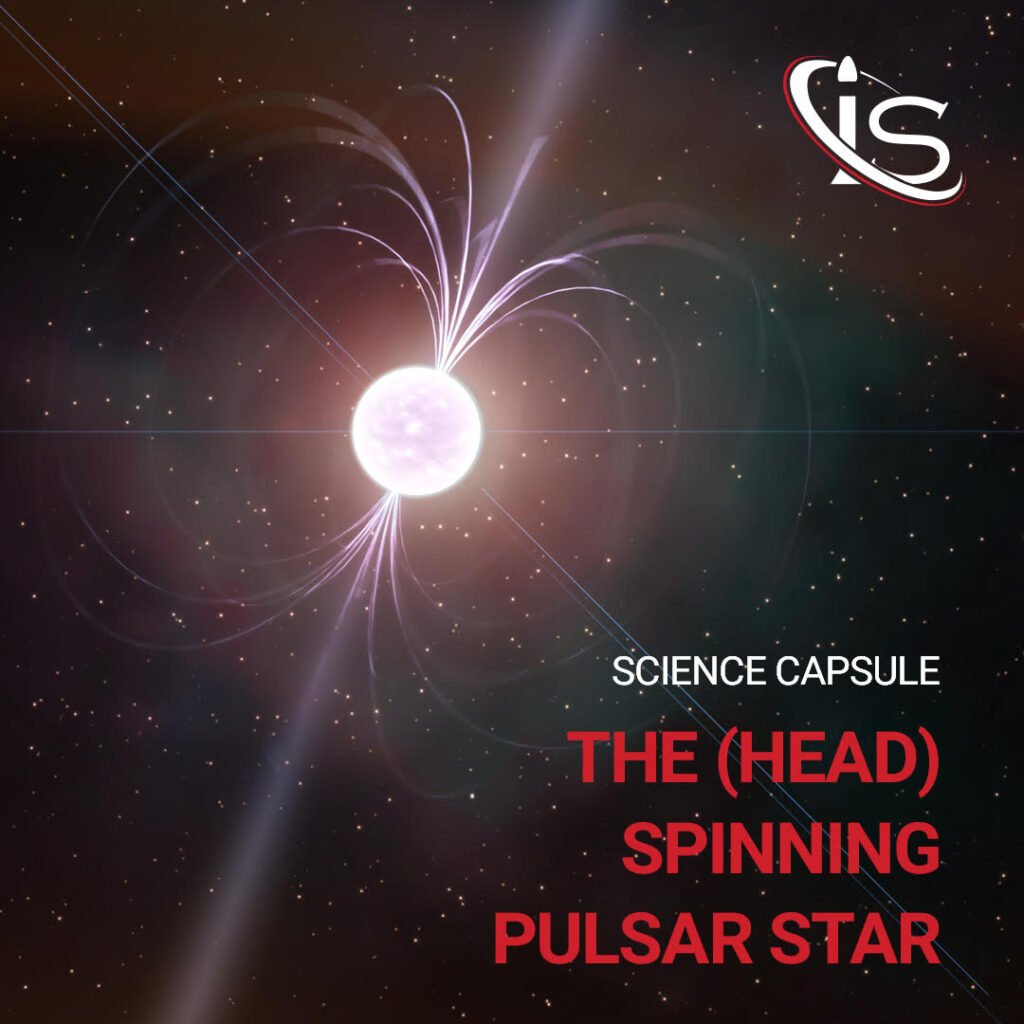
Just Add Rotation
For anyone wondering why I used the phrase above to introduce this topic, the answer is twofold. One, that is why pulsars appear to intermittently emit light. Two, my favorite activity in the world is ballroom dancing and, both for that and stars, adding rotation always makes things more fun. So, how exactly does a Pulsar rotating affect the way it sends out light? Well, it does not. Not really at least.
What is actually happening is that the Pulsar is constantly emitting two beams of light in opposite directions. These beams are special in and of themselves, and we will get to this soon, and are relatively narrow. Because of this, as the Pulsar rotates, a faraway observer will only see the beams at certain intervals. This gives off the impression that the (dead) star is only emitting light at certain times. Basically, a Pulsar functions in a similar way to a lighthouse.
How Does a Pulsar Rotate?
Different Pulsars will have differing rates of rotation, but they can generally be classified in two categories: “Slow” and “Millisecond” Pulsars. Slow Pulsars are usually rotating in the order of 1 rotation per second. They are also the most common of the two types. Millisecond Pulsars rotate, as many of you probably have guessed, in the order of hundreds of times per second. So far, only around 200 Pulsars, of the total 2000 discovered up until now, have fallen under this category. The fastest ones have recorded spinning frequencies of 700+ rotations per second.
Why Are Pulsars Rotating So Fast?
Now the frequency of these rotations might seem very high, but there is actually a very simple physics-related explanation. These Pulsars all start off as regular stars, albeit on the more massive side. That means that they were already rotating before undergoing the various stages of a star’s end state that led to the formation of the Pulsar. Therefore, once the mass gets condensed into such a small space, literally hundreds of thousands of times smaller than the original star, the angular velocity has to massively increase to compensate. After all the formula for angular velocity is as follows:
Where:
: Angular Velocity
v : Tangential/Linear Velocity
r : Radius
This is why we can spin much faster on ourselves with our arms close to us, as opposed to extended outwards.
Going back to the Millisecond Pulsars, and the way scientists believe they form is by basically zapping momentum and energy from another nearby star. The way this would happen is gradual. The Pulsar would start to siphon the momentum and matter/energy of the second star, thus increasing its own spin rate. This would eventually lead to the second star being “consumed” by the Pulsar. Scientists also believe this to be the reason why Millisecond Pulsars have never been found with nearby companion stars.
Why Does a Pulsar Star Radiate Light?
As I said before, the light coming off of a Pulsar is quite peculiar. First, a Pulsar can radiate light in all frequencies, from radio waves to gamma-rays. Second, the two narrow beams of light that characterize it are in the radio waves end of the electromagnetic spectrum. Furthermore, they are coherent beams of light, similar to the light of a laser. As such, they will appear a lot brighter with the same amount of energy than light coming from a diffused source.
Unfortunately, the exact way in which a Pulsar functions is still not completely understood. What is known, however, is that the combination of the Pulsar’s magnetic field and rotation are key for its emissions. This is because of the way magnetic fields are related to electric fields.
As a magnetic field spins, moves, or changes in any way, a resulting electric field will appear. Therefore, as Pulsars spin, their massive magnetic fields will begin producing matching electric fields. Because of this, any charged particles inside the Pulsar’s electromagnetic field will experience an electromagnetic force that will accelerate them through space. This will, in turn, lead to the emission of corresponding light particles.
Based on the magnitude of the Pulsar’s electromagnetic field, light in the optical, UV, and X-rays range could be produced in the Pulsar’s magnetosphere. As for the light in the gamma-rays range, scientists have observed that it is produced in a separate region of space surrounding the Pulsar. Unfortunately, it is still not clear how exactly Pulsars produce light in the gamma-rays frequencies. In fact, scientists do not fully understand the mechanism behind the emission of the radio waves either. But, in a way, not having the “full picture” for Pulsars makes them even more intriguing to study.